High Frequency VCO Design and
Schematics
PDF High Frequency VCO POBIERZ (89 KB)
This note will review the process by which VCO (Voltage Controlled
Oscillator) designers choose their oscillator’s topology and devices
based on performance requirements, components types and DC power
requirements.
Basic oscillator design specifications often require a given output
power into a specified load at the design frequency. The drive level
and bias current set the fundamental output current and the
oscillation frequency is set by the resonator components.
Transistor selection of the transistor should consider noise,
frequency, and power requirements. Based on the particular device,
the design may account for parasitics of the device affecting
resonator components as well as nonlinear performance
specifications.
All the VCO schematics presented below were practical build using
the Infineon SiGe transistor BFP420, and any of them can be re-tuned
for different frequency ranges changing varicaps and LC tank values.
VCO Specifications
The VCO must exhibit a low Phase Noise in order to meet the
Sensitivity, Adjacent Channel and Blocking requirements. In digital
modulation scheme the VCO’s Phase Noise affects the Bit Error Rate
requirements. High Pushing (change of the oscillation frequency with
supply voltage) can cause Phase Noise degradation due to increased
sensitivity to the power supply noise.
Phase Noise varies typically by 3dB with temperature, in the –55ºC
to +85ºC range.
A buffer at the output is necessarily to isolate the VCO from any
output load variations (Pulling) and to provide the required output
power. Meeting simultaneously the output power and load pull
specification directly with a stand-alone oscillator would be
difficult. However, this buffer amplifier requires a higher supply
current. Alternative would include to use at the output circulators,
isolators or passive attenuators.
VCO output power is usually measured into a 50 ohm load. Output
power requirements specified in dBm, and tolerances vs tuning
frequency in ± dB.
The tuning slope is the slope of the frequency to voltage tuning
characteristic at any point and is the same as modulation
sensitivity. The slope could be positive or negative. For a positive
slope, the output frequency. increases as the tuning voltage
increases. Similarly for a negative slope, the output frequency
decreases as the tuning voltage increases
A monotonic tuning characteristic means that the frequency is single
valued at any tuning voltage and that the slope has the same sign
across the tuning range.
Tuning sensitivity as a function of tuning voltage is a measure of
tuning linearity. For any given application, have to specify the
minimum and maximum of the tuning sensitivity. In the case of a VCO,
the frequency coverage is rather restricted since the influence of
the feedback network is small compared to the active device itself.
Conventional oscillator designs (with a LC circuit or
transmission-line equivalent coupled to a negative-resistance active
device will only provide a restricted frequency coverage and poor
stability). A negative resistance can easily be obtained from most
microwave transistors when considering chip and package parasitics.
Tuning flatness - As the VCO frequency range is increased, the
difficulty to achieve a flat output power is increased. Adding an
output filter to suppress harmonics may in some cases degrade power
output flatness.
The drive level should consider the trade-off between harmonic
content, oscillator stability, and noise.
In order to lower the VCO Phase Noise, a number of rules should be
respected:
The active device has noise properties which generally dominate the
noise characteristic limits of an oscillator. Since all noise
sources, except thermal noise, are generally proportional to average
current flow through the active device, it is logical that reducing
the current flow through the device will lead to lower noise levels.
Narrowing the current pulse width in the active device will decrease
the time that noise is present in the circuit and therefore,
decrease Phase Noise even further.
Maximize the loaded Q of the tuned circuit in the oscillator.
There is a trade-off between the Q factor of the oscillator, its
size and its price. The low Q-Factor of an LC tank and its component
tolerances needs careful design for phase noise without individual
readjustment of the oscillators.
Usually a larger resonator will have a higher Q (e.g. a quarter
wavelength coaxial resonator).
Choose an active device that has a low flicker corner frequency.
A bipolar transistor biased at a low collector current will keep the
flicker corner frequency to a minimum, typically around 6 to 15 KHz
(Most semi-conductor manufacturers can provide the frequency corner
(fc) of their devices as well as the 1/f characteristic.
Maximize the power at the output of the oscillator.
In order to increase the power at the input of the oscillator, the
current has to be increased. However, a low current consumption is
critical to preserving battery life and keeping a low fc. In a
practical application, the current will be set based on output power
required to drive the system (typically a mixer), and then the Phase
Noise will need to be achieved through other means.
Choose a varactor diode with a low equivalent noise resistance.
The varactor diode manufacturers do not measure or specify this
parameter. The best approach is then empirical; by obtaining
varactors from several vendors and experimentally finding out which
one yields the lowest phase noise in the VCO circuit and thus has
the lowest equivalent noise resistance.
There are two basic types of varactors: Abrupt and Hyperabrupt.
- The abrupt tuning diodes will provide a very high Q and will also
operate over a very wide tuning voltage range (0 to 60 V). The
abrupt tuning diode provides the best phase noise performance
because of its high quality factor.
- The hyperabrupt tuning diodes, because of their linear voltage vs.
capacitance characteristic, will provide a much more linear tuning
characteristic than the abrupt diodes. These are the best choice for
wide band tuning VCO's. An octave tuning range can be covered in
less than 20 V tuning range. Their disadvantage is that they have a
much lower Q and therefore provide a phase noise characteristic
higher than that provided by the abrupt diodes.
Keep the voltage tuning gain (Ko) to the minimum value required.
This is the most challenging compromise because the thermal noise
from the equivalent noise resistance of the varactor works together
with the tuning gain of the VCO to generate phase noise. This
compromise will be the limiting factor determining the phase noise
performance.
Noisy power supplies may cause additional noise. Power supply
induced noise may be seen at offsets from 20 Hz to 1 MHZ from the
carrier. If the VCO is powered from a regulated power supply, the
regulator noise will increase depending upon the external load
current drawn from the regulator. The phase noise performance of the
VCO may degrade depending upon the type of regulator used, and also
upon the load current drawn from the regulator. To improve the phase
noise performance of the VCO under external load conditions it is
always a good design philosophy to provide RF bypassing of power and
DC control lines to the VCO. RF chokes and good bypassing capacitors
(low ESR) is recommended at the DC supply lines. This will minimize
the possibility of feedback between stages in a complex subsystem.
Improved bypassing may be provided by incorporating an active filter
circuit.
VCO Topologies
Parallel Tuned Colpitts VCO
There are 3 types of BJT Colpitts VCOs. Common-Collector,
Common-Emitter and Common-Base.
The most used is Common-Collector configuration where the output is
often taken from the collector terminal, simply acting as a buffer
for the oscillator connection at the base-emitter terminals.
This is the only Colpitts arrangement in which the load is not part
of the three-terminal model or the oscillator equation; though care
must be taken to ensure that the collector output voltage does not
significantly feedback through the base-collector junction
capacitance.
As an alternative, the output of the common collector could also be
taken across emitter resistance Re.
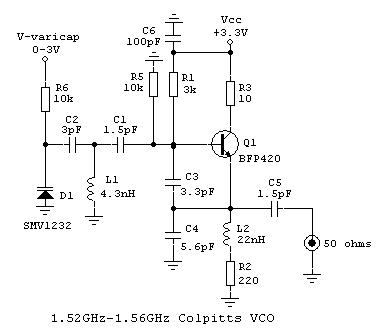
The ratio of the feedback capacitors in the Colpitts VCO (C3 and
C4), is more important than the capacitor’s actual values. A good
place to start is with a one to one ratio. The loaded Q of the
resonator circuit can be increased by reducing C3 or increasing C4.
Doing so however, reduces the loop gain in the oscillator, and
enough loop gain must be maintained to guarantee oscillation
start-up under all conditions (mainly under different temperatures
and system output loads).
The value of the collector resistor, R3 affects the oscillator loop
gain. As in a common collector amplifier, the lower the impedance in
the collector circuit the more loop gain the circuit will have. This
resistor provides another means of controlling the loop gain of the
oscillator since a good oscillator design has just enough loop gain
to guarantee reliable oscillation start-up. If there is to much loop
gain the oscillator will operate in deep compression which will load
the Q of the resonator circuit because the input impedance at the
base of the transistor is very low when current saturation occurs.
The resistor also tends to minimize the level of the harmonics.
L2 is chosen as an RF choke to provide a high impedance in the
emitter circuit and ensure that most of the oscillator power is fed
back to the base of Q1 instead of being dissipated in R2.
Emitter resistor R2 is used for current feedback thus providing a
stable DC bias point that will be independent of the beta of the
transistor.
C1 capacitor defines the amount of coupling between the active
device and the resonator. The lighter the coupling (a smaller value
of C1), the better the loaded Q of the resonator is, which results
in a better phases noise performance. However, the compromise is a
reduced output power and the potential for the VCO not to start
under all operating conditions (especially at higher temperatures
when current gain is reduced). Designing the system with too light
of a coupling may also results in a sensitive design which may yield
potential manufacturing problems.
The final tuning component of the oscillator, C2 sets the voltage
tuning gain of the oscillator. This capacitor should keep the
coupling as light as possible while maintaining the required
frequency tuning range of the VCO so that the varactor’s phase noise
contribution is reduced to a minimum. If the coupling is too light,
the oscillator may not start under certain conditions. The worst
case condition for this oscillator topology is when V-varicap is set
at zero volts.
A good way to check if C2 is large enough for reliable oscillator
start up is to monitor the output power of the VCO with zero volts
on the tune line. The power with V-varicap at 0V should be within 1
dB of the power with V-varicap at 3V. If C2 is too small, the output
power of the VCO will fall off sharply when V-varicap approaches
zero volts or the oscillator may stop completely.
One good reason to use a transistor with a high Ft such as the
BFP420 (Ft = 25GHz) is that C2 can be small and oscillation start-up
will be reliable simultaneously.
In order to ensure that the loaded Q of the resonator circuit is not
the limiting factor in phase noise performance, the varactor can be
replaced with a fixed 2.5pF capacitor and compare the results. A
varactor can degrade up to 5-6dB
The varactor can reduce the Q of the resonator circuit but this
effect is secondary to the varactor modulation due to its own
equivalent noise resistance. One way of reducing this effect is to
parallel two or more varactors of smaller value while keeping the
same tuning curve. This effectively reduces the equivalent noise
resistance.
Series Tuned Colpitts VCO (Clapp VCO)
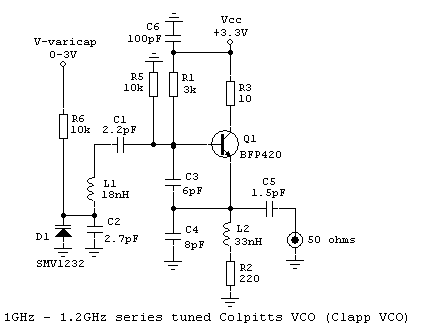
The series-tuned Colpitts circuit (or Clapp oscillator) works in
much the same way as the parallel one.
The difference is that the variable capacitor, C1, is positioned so
that it is well-protected from being swamped by the large values of
C3 and C4.
In fact, small values of C3, C4 would act to limit the tuning range.
Fixed capacitance, C2, is often added across the varicap to allow
the tuning range to be reduced to that required, without interfering
with C3 and C4, which set the amplifier coupling.
The series-tuned Colpitts has a reputation for better stability than
the parallel-tuned original. Note how C3 and C4 swamp the
capacitances of the amplifier in both versions.
The oscillation frequency is given by: ω2 L =
[1/(C2+Cvar)]+(1/C3)+(1/C4)
Wideband Colpitts VCO
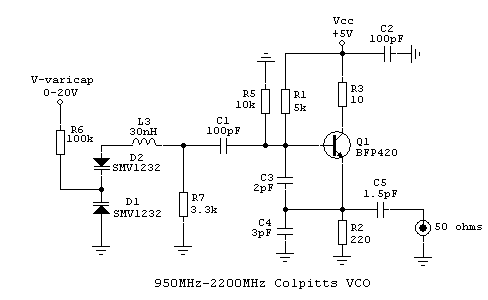
This wideband Colpitts VCO uses a series back-to-back connection of
two SMV1232 varactors instead of a single varactor. This connection
allows lower capacitance at high voltages, while maintaining the
tuning ratio of a single varactor. The back-to-back varactor
connection also helps reduce distortion and the effect of fringing
and mounting capacitances.
The wideband Colpitts feedback capacitances C3, C4 were optimized to
provide a flat power response over the wide tuning range. These
values may also be re-optimized for phase noise if required.
The circuit is very sensitive to the transistor choice (tuning range
and stability) due to the wide bandwidth requirement.
DC bias is provided through resistors R6 and R7, which may affect
phase noise, but allows the exclusion of RF chokes. This reduces
costs and the possibility of parasitic resonances which is the
common cause of spurious responses and frequency instability.
Hartley VCO
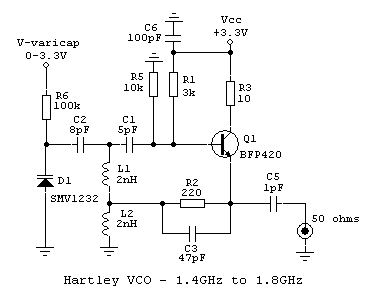
The Hartley VCO is similar to the parallel tuned Colpitts, but the
amplifier source is tapped up on the tank inductance instead of the
tank capacitance. A typical tap placement is 10 to 20% of the total
turns up from the “cold” end of the inductor. (It’s usual to refer
to the lowest-signal voltage end of an inductor as cold and the
other, with the highest signal voltage as hot.). The same as in
Colpitts case a good place to start is with a one to one ratio.
C2 limits the tuning range as required.
C1 is reduced to the minimum value that allows reliable starting.
This is necessary because the Hartley’s lack of the Colpitts’s
capacitive divider would otherwise couple the transistor
capacitances to the tank more strongly than in the Colpitts,
potentially affecting the circuit’s frequency stability.
Wideband Differential Push-Push VCO
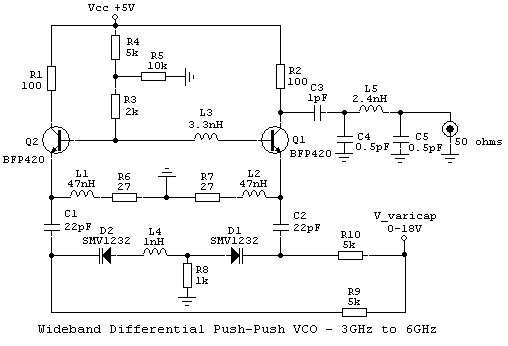
The circuit schematic shows a pair of transistors in a single
feedback loop, connected so that collector currents would be 180°
shifted. A pair of back-to-back connected SMV1232 varactors is used
to allows lower capacitance at the high voltage range, without
changing the tuning ratio.
Varactor DC biasing is provided through resistors R8, R9 and R10,
which may affect the phase noise, but eliminate the need for
inductive chokes. This eliminates the possibility of parasitic
resonances that could affect the wide tuning range and also cause
for frequency instability and spurs.
The DC chokes, L1 and L2 are used for phase correction between pairs
and their losses is dominated by the series emitter resistors R6 and
R7.
The DC blocking series capacitances C1 and C2, including associated
parasitics, shall have the SRF outside of the tuning range.
A three-pole Low Pass Filter at the output is used to filter the
unwanted spurious.
Differential Cross-Coupled VCO
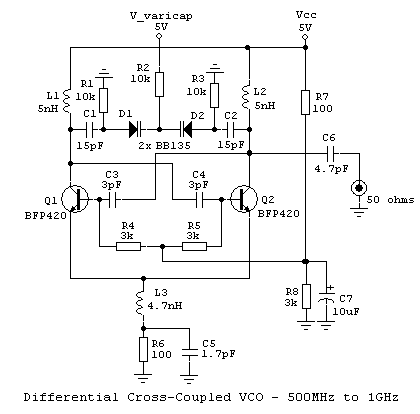
The cross-coupled differential transistor pair presents a negative
resistance to the resonator due to positive feedback.
This negative resistance cancel the losses from the resonator
enabling sustained oscillation.
Frequency variation is achieved with two varicap diodes BB135.
An optimal trade-off between thermal noise- induced phase noise and
DC power dissipation can be achieved when the oscillation amplitude
is designed to set the differential pair transistors to operate at
the boundary between saturation and linear regions.
The excess noise factor F is dominated by the noise from the tail
current source near even harmonics of the carrier frequency. In
order to improve phase noise this contribution has to be minimized.
An efficient way of doing this is to use a noise filtering
technique. An inductor L3 and capacitor C5 forms a 2nd order
low-pass filter which prevents noise at even harmonics from being
injected into the feedback path of the oscillator.
The noise filter leaves low-frequency noise from the tail current
source unaffected. Low-frequency noise from the tail current source
is also up-converted to the carrier as amplitude modulation.
Low-frequency noise on the tuning line modulates the non-linear
capacitance of the varactors giving rise to phase noise variation
with control voltage.
The phase noise degradation due to control voltage noise is very
significant at the lower tuning range where the varactors are most
non-linear. The stack of two varactors reduces the varactor gain
Kvco at the lower tuning range which in turn reduces phase noise
variation with control voltage.
Negative Resistance VCO
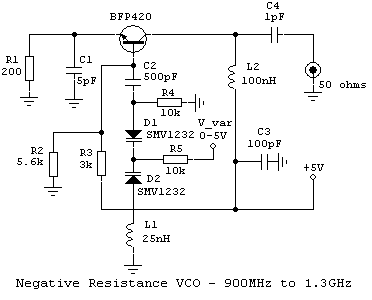
The resonator of the Negative Resistance VCO is a series-tuned base
network consisting of two series varicap capacitances and an
inductor for the positive reactance element.
Performance is highly dependent on the transistor type. Certain
component values are critical.
This oscillator actually works best when lower Ft transistors are
used. The circuit can be envisioned as a series-tuned Clapp, with
internal transistor base-to-emitter capacitance and
collector-to-emitter capacitance acting as a voltage divider.
Microwave transistors with little internal capacitance do not work
well except at the high end of the useful range of this oscillator
type. Higher Ft devices required increased capacitance added at the
emitter. At the low end of the frequency range, adding external
base-to-emitter capacitance is sometimes necessary,
If bias conditions result in a emitter resistance below about 200
ohms, an RF choke may be required in series with the resistance.
This choke must be free of any resonances in the operating frequency
range.
The output can be taken from several points. The L1 inductor can be
tapped. As the tap is moved toward the transistor, more power is
coupled out. If the tap is too close to the transistor, the loading
reduces the oscillation margin, and the operating frequency becomes
more load dependent.
The output can be taken by capacitive coupling at the emitter (low
level) or at the collector (higher level, but have more spurious).
Because the negative resistance oscillator uses a series-tuned
resonator, the varactors lead inductance becomes a part of the
resonator. This is an advantage over varactor-tuned oscillators
using parallel resonators. The base coupling capacitor inductance
and transistor base inductance are also absorbed.
The loaded Q of negative resistance oscillators is typically less
than 5 and this circuit defies attempts at improving the Q. When
used as a broadband varactor-tuned VCO, the low loaded Q does not
limit phase noise performance significantly because varactor
modulation noise predominates, particularly at higher offset
frequencies.
Franklin VCO
Franklin oscillator uses two transistor stages having the same
common terminal (emitter for bipolar device) when the greater power
gain and better isolation from the resonant circuit is possible
compared with the case of a single-stage configuration.
There are two possible configurations for the resonant circuit,
parallel and series. The circuit presented below uses a parallel LC
resonant circuit (L1 and the varctor diode).
In the case of a parallel resonant circuit configuration, the
resonant LC circuit is isolated from the input of the first stage
and the output of the second stage by means of small shunt
capacitances C1 and C2 having high reactances at the resonant
frequency.
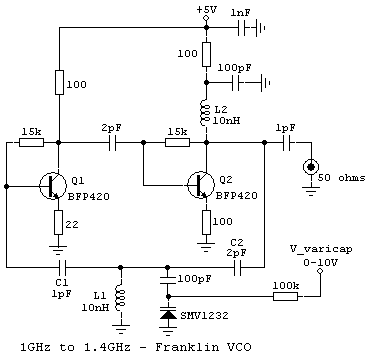
In this circuit, each stage shifts phase 180° so that the total
phase shift is 360° which is equivalent to zero phase shift. We may
say that one stage serves as the phase inverting element in place of
the RC or LC network which generally performs this function. It is,
from an analytical viewpoint, immaterial which stage we choose to
look upon as amplifier or phase inverter. The configuration is
essentially symmetrical in this respect; both stages provide
amplification and phase inversion.
The salient feature of the Franklin oscillator is that the
tremendous amplification enables operation with very small coupling
to the resonant circuit.
Therefore, the frequency is relatively little influenced by changes
in the active device, and the Q of the resonant circuit is
substantially free from degradation.
The closest approach to the high frequency stability inherent in
this oscillator is attained by restriction of operation to, or near
to, the Class-A region. This should not be accomplished by lowering
the amplification of the two stages, but, rather by making the
capacitors C1 and C2 very small.
Additionally, a voltage-follower 'buffer' stage is helpful in this
regard. Extraction of energy directly from the resonant tank, would,
of course, definitely negate the frequency stability otherwise
attainable.
Obviously, the Franklin oscillator is intended as a low-power
frequency-governing stage, not as a power oscillator.
Goral VCO
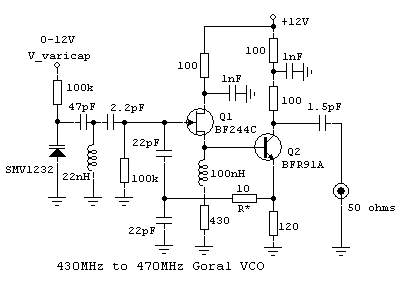
The Goral VCO has an emitter-follower stage inserted in the feedback
path of an otherwise conventional Colpitts oscillator circuit.
The midpoint of the capacitive divider (which is actually part of
the resonant tank) now sees a much lower impedance with respect to
ground than would be the case without the emitter follower.
Because the feedback gain of a Goral VCO is greater than a standard
Colpitts, placing a feedback resistor R*, helps improving the
overall phase noise performance and improving also the linearity,
reducing the VCO generated harmonics.
The power gain of the JFET/BJT combination is much greater than that
of the JFET 'oscillator' alone. There is latitude for considerable
experimentation in the ratio of the two capacitors used in the
Colpitts section of the circuit. This ratio can be optimized for
frequency stability without easily running out of feedback.
Note that the emitter-follower is directly coupled to the JFET. It
may be necessary to experiment with bias-determining resistances to
ascertain Class-A operation from the emitter-follower. Also, the
output transistor is intended to operate in its Class-A region.
Cascode VCO
To provide higher isolation of the load from the VCO resonant
circuit a cascode VCO configuration, can be used.
The negative resistance oscillation conditions for common emitter
transistor Q1 are provided by using the feedback inductance L1.
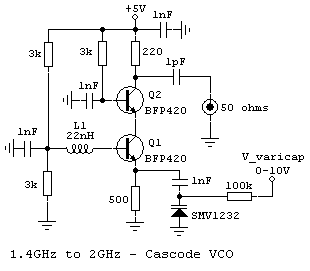
Vackar VCO
And here is the winner. If you want to build a very stable, low
phase noise, and low spurious VCO, definitely Vackar VCO is the
choice.
This is not a common type in the RF “professional” world, one reason
could be the name of its inventor.
A Vackar VCO is a variation of the split-capacitance oscillator
model. It is similar to a Colpitts or Clapp VCO in this respect. It
differs in that the output level is more stable over frequency, and
has a wider bandwidth when compared to a Colpitts or Clapp design.
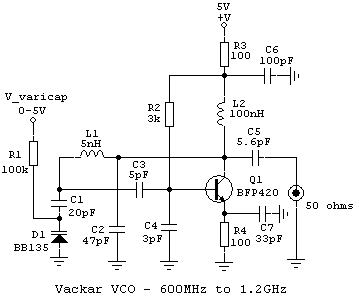
The Vackar VCO circuit incorporates a π-section tank to attain the
needed 180° phase-reversal in the feedback loop.
However, the inverted feedback signal is not directly fed back to
the input of the active device; rather, it is loosely coupled
through a small capacitor. Often, a shunt capacitor is introduced to
further reduce the coupling.
The basic idea is to isolate the resonant circuit as much as
possible from the input of the active device, consistent with
obtaining reliable oscillation.
This circuit is particularly advantageous with solid-state devices,
and especially with bipolar transistors that have inordinately-low
input impedances and that present a widely-varying reactance to the
tuned circuit as a consequence of temperature and voltage changes.
Once the overall circuit is operational, the values of capacitance
C1 in series with Cvar and collector capacitance (C2) may be
optimized for best stability. Generally, it will be found that the
capacitor closest to the collector of the transistor can be several
times larger than the capacitor associated with the base circuit.
The introduction of attenuation in the feedback loop (via the small
capacitor in the Vackar) prevents over-excitation and effectively
isolates the resonant circuit from the active device.
The frequency tuning range of Vackar VCO is above one octave, not
observable to many oscillator types.
The frequency tuning is provided independently of the coupling to
the LC tank circuit.
The parametric variables of the transistor (which depends by the
bias current and temperature), are isolated from the resonator.
The transistor input is not overloaded as other VCO circuits and the
collector output has low impedance providing low gain just to
maintain the oscillation.
The feedback division ratio is fixed (typical range for coupling
ratio is 1:4 up to 1:9). Even if the VCO is tuned, the impedance
divider is fixed, in this way increasing the stability.
Two negative sides of Vackar VCO are the critical starting
oscillation point, and the low output level, which always requires
to use a buffer amplifier. When the oscillation doesn’t start means
that it doesn’t have enough positive feedback, as for to begin the
oscillation and maintain it in the time. In the above schematic C3
and C4 are critical values finding this point.
L2 is used as an RF choke with SRF outside of the frequency range,
to don’t affect the tuning range and flatness over frequency. It is
important that the RF choke in the collector circuit 'looks good' at
the operating frequency (presents a high inductive reactance).
Resonances from distributed capacitance in the choke windings,
especially those in the series-resonant mode, can degrade stability
or even inhibit oscillation. Ferrite-core chokes are generally
suitable for this application. Sensitivity to RF choke
characteristics is common to all oscillator circuits that use chokes
for shunt-feeding the DC operating voltage to the oscillator.
References:
1. Alpha Industries - VCO Application notes
2. Minicircuits - VCO Application notes
3. Oscillator Basics and Low-Noise Techniques for Microwave
Oscillators and VCOs - U.Rohde
4. Oscillator Design and Computer Simulation - R.Rhea
5. RF and Microwave Transistor Oscillator Design – A. Grebennikov
6. Practical Oscillator Handbook - I. Gottlieb
7. RF Design Magazine - 1997 - 2003
8. Microwave Journal - 1997 - 2008
9. Microwaves & RF - 2002 - 2006 |